Vascular plants, unlike non-vascular plants, have specialized tissues for transporting water with both inorganic and organic dissolved substances. These tissues are known as vascular tissues, which include the xylem and phloem. Vascular tissues emerged around 450 million years ago during evolution, when plants invaded the land. Lycohpytas were the first to show vascular tissues. These tissues allowed plants to connect the two main domains of the land plants: the aerial part, which performs photosynthesis to make organic molecules, and the underground parts, which are in charge of fetching water and minerals. The xylem is specialized in transporting water, inorganic compounds, and some organic substances from roots to other plant organs, while the phloem mostly transports organic substances synthesized in plant body organs, such as leaves and storage tissues, toward the rest of the plant. More than 300,000 species of vascular plants are currently known.
1. Introduction
Vascular tissues perform three main functions in plants. a) Physiologically, plants need vascular tissues to increase their size by distributing water and organic substances to feed the cells. Although almost each plant cell may exchange substances with the adjoining cells through plasmodesmata, which are pores that directly communicate two cytoplasms across the shared cell wall of neighboring cells, this is a short-distance transport that only reaches a few cells away. Thus, for long-distance transport, a specialized tissue is needed: the vascular tissue. b) Vascular tissues also have a mechanical role in supporting the aerial parts and giving consistency to the underground organs, acting as a skeleton. c) Another function of vascular tissues is to allow communication between distant organs of the plant body by transporting meaningful molecules, like some phytohormones, ARNs, proteins, and so on.
Vascular tissues arise during embryonic development, before germination. During this period, provascular cells are differentiated (4 provascular cells in A. thaliana) between the epidermis and the ground tissue. Provascular cells become the procambium meristem in the caulinar and root apical meristems, which gives rise to the primary vascular tissue. The formation of primary xylema and phloem happens after germination. Some cells of the apical meristem may also differentiate directly into vascular cells. The primary vascular tissues are protoxylema and protophloem, which are later replaced by metaxylem and metaphloem as the main functional conducting tissues during organ maturation. Metaxylema and metaphloem differentiate from the procambium meristem too. If secondary growth takes place in the plant, the secondary xylem and secondary phloem are formed from the vascular cambium meristem, while the metaphloem and metaxylem become nonfunctional. At the same time, the caulinar and apical meristems continue to produce procambium (primary vascular tissues) as the stem and roots grow in length.
There is a longitudinal and a transversal organization of the vascular tissues, although it varies with the type of organ, plant age, and plant species. The basic organization of the vascular tissues is under the control of auxin and citokinin hormones. Several types of cells form the vascular tissues, some of which have been key elements for evolutionary studies. Most of these cell types derive from the same meristematic cell, which is why xylem and phloem are physically close.
Stele
The set of vascular tissues of a shoot or root is known as the stele. The type of stele depends on the species, branching pattern, leaf disposition, gradient and concentration of auxin, and everything is under the influence of the genome. The auxin released from leaves primordia in the stem tip determines the disposition of new leaves and new vascular bundles, since the auxin influences the differentiation of primary vascular tissues.
The stele is named according to the organization of the vascular tissues (Figure 1). When the vascular tissues form a cylinder without medullary parenchyma, the estele is named the protoestele. If the core of the cylinder is medullary parenchyma, the stele is known as a siphonostele. An eustele is a type of siphonostele where the wall of the cylinder shows discontinuities and is formed of many vascular bundles. The eustele is typical of dicots with primary growth and monocots at their stem apical zones. In monocots, the eustele is transformed into an atactostele by branching the vascular bundles. The atactostele is typical of monocots. If we attend to the location of xylem and phloem, there are different types of organization (Figure 1). In the radial organization, the xylem and phloem alternate. The collateral organization is found in angiosperms and gymnosperms and consists of many vascular bundles, each of which is composed of xylem and phloem. It can be open collateral if the procambium is conserved between the xylem and phloem, or closed collateral when the meristem is lost. In the bicollateral organization, the xylem is covered by the phloem, which can be found in some angiosperms. There are two types of organization with concentric vascular tissues: the amphivasal (with inner phloem) is often observed in monocots and some dicots, whereas the amphicribal (with inner xylem) is found in ferns and some aquatic angiosperms.
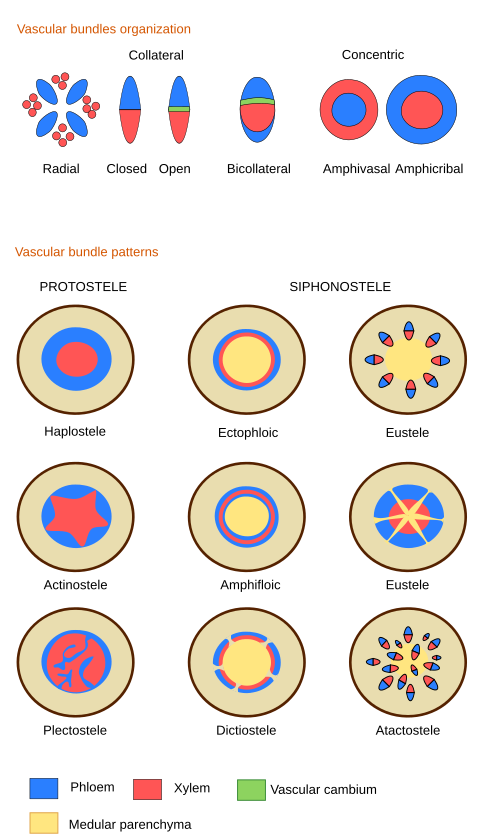
Vascular tissue arrangements different from eusteles during the primary growth of organs are collectively known as vascular variants or alternative growth patterns. For instance, some species develop vascular bundles in the medullary parenchyma combined with several rings of procambium that yield rings of vascular tissues. It is known as the polycyclic stele. Some of these species produce phloem among the secondary xylem (see below), which is grouped in the so-called phloematic islands or interxylar phloem. There are other organizations with vascular tissues forming compartments as independent elements along the shoot. These shoots are referred to as composed shoots.
In angiosperm leaves, the xylem is usually toward the adaxial surface (upper) and the phloem toward the abaxial one (lower). The vascular tissues are organized in cords, which are parallel in monocots and branched in dicots.
2. Xylem
The xylem is responsible for transporting and distributing water and mineral salts, which mainly come from the root, to the plant body. It also transports some organic and signaling molecules. Furthermore, it is the main tissue for mechanical support of plant organs, particularly during secondary growth. The wood of trees and some plants is largely xylem.
Four cell types are present in the xylem: a) vessel elements and b) tracheids are the conducting cells, also known as tracheary elements; c) parenchyma cells work as storing and communication cells; and d) sclerenchyma cells and sclereids are supporting cells (Figure 2).

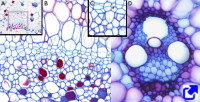
In gymnosperms, the xylem mostly consists of two cell types: tracheids and parenchyma cells. Tracheids are up to 90 % of the xylem, with two main functions: conducting and mechanical support. Parenchyma cells have roles in storing and communicating. In angiosperms, the xylem is more complex. The vessel elements are conducting and supporting cells, although tracheids are also present; sclerenchyma cells and sclereids provide mechanical support; and parenchyma cells are storage and communicating cells.
Tracheary elements (a and b) (Figures 2 and 3) are cells containing lignin in their thick and hard secondary cell walls. These cells lose their cytoplasmic content during differentiation. The secondary cell wall of the tracheary cells forms different thickenings depending on the cell type and developmental stage of the organ. Thus, vessel elements and tracheids are identified at light microscopy by the morphology of these thickenings, which can be annular, helical, scaliform, reticulated, or dotted structures. The annular thickenings are observed in the protoxylem, which are replaced by helical thickenings. Metaxylem shows scaliform, reticulated, and dotted patterns (Figure 4). The scaliform pattern is often observed in pteridophytes but is not frequent in seed plants. The morphology of the thickenings is determined by the orientation of the cellulose fibers in the cell wall, which in turn depends on the arrangement of the microtubules beneath the plasma membrane. The name "trachea" was given by M. Malpighi because the tracheary elements resemble the respiratory tracheas of insects. Forming a tracheary element is a process with several steps: a cambium meristematic cell divides periclinally, the new cell grows in length, cellulose and hemicellulose are lied to form the secondary cell wall, lignin is synthesized and released into the cell wall, and regulated cell death occurs. This process is similar in angiosperms and gymnosperms, but the times and components may be different.
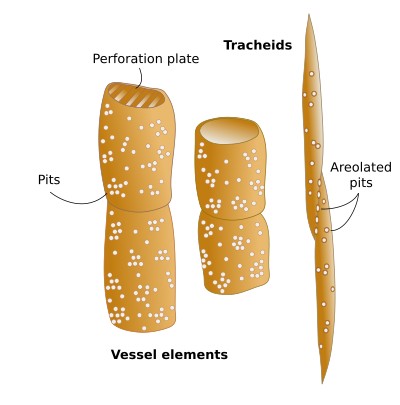
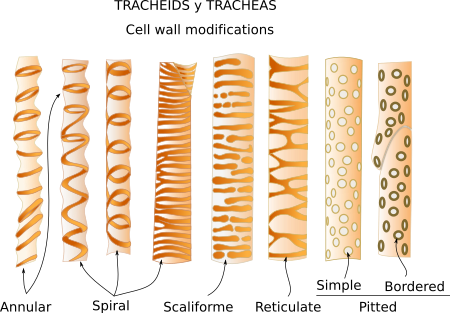
Regulated cell death happens in many cells through the plant organs, such as leaves during senescence, roots during the formation of aerenchyma, flowers during development, and seeds during germination. The degradation of the cell content is fast in the tracheary cells (but slower in sclerenchyma tissues). During xylem differentiation, several factors appear to trigger the process, such as nitric oxide. It is interesting that most cells die after performing their functions, but the conducting cells of the xylem perform their functions once they are dead. Regulated cell death is a fast process that lasts a few minutes and starts with a massive influx of calcium and the breakage of the vacuole membrane, which release many hydrolytic enzymes into the cytoplasm. The previous storage of these enzymes in the vacuole indicates that the process was initiated much earlier. The dead process runs parallel to the formation of the secondary cell wall. In two days, the cell will be empty and ready for conduction.
Vessel elements (a) are cells with a larger diameter and more flat ends when compared to tracheids (Figures 2, 3, and 5). They are connected longitudinally to one another to form long tubes, also known as vessels. Water is conducted through the symplastic pathway, that is, within the cells. The water crosses from one cell to the next through the perforation plates, which are the specialized perforated transverse cell walls at both ends of each cell. Furthermore, water can cross the lateral walls of the vessels through pits and pass to the lateral adjoining cells of the xylem. Vessel elements are the main conducting cells of the xylem of angiosperms. The vessels (the rows) are 1 cm to 1 m long and 15 to 300 µm wide. The vessel elements (the individual conducting cells) may not grow larger than the meristematic cell they are differentiated from, but the diameter is usually larger.
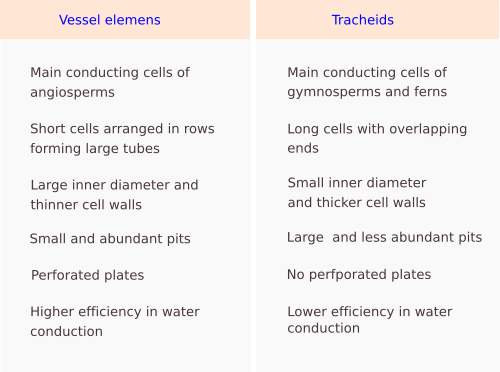
Tracheids (b) are the other conducting cell type of the vascular plants. They are elongated, fusiform, and thin (Figure 3). It is the only tracheary element in pteridophytes and gymnosperms. In gymnosperms, tracheids are up to 90% of the total xylem cells, and, in addition to conduction, they are the main mechanical supporting cells. To perform these functions, they have to die. In gymnosperms, tracheids are long and tipped, 6 mm long and 6 to 60 µm wide. In transverse sections, they are round to polygonal. Angiosperms have both tracheids and vessel elements. Water is conducted intracellularly and crosses from one cell to the next by symplastic transport through areolate pits found at the cell walls of both ends of each tracheid, which overlap with those of the previous and following cells, respectively (Figure 3). Water is also laterally transported through areolate pits located at the lateral cell walls. Because tracheids do not have perforation plates, they have a lower capacity for water conduction than vessel elements. Furthermore, tracheids have thicker cell walls and a smaller inner volume than vessel elements. Conifers show tracheids with very large and round areolate pits and an inner structure known as the torus, which is an oval thickening of the cell wall. The torus can regulate the flow of water through the pit.
From an evolutionary point of view, tracheids are older than the vessel elements. That is, tracheids were the only conduction cell type in the first vascular plants, and the vessel elements were "invented" later. It is also thought that both the vessel elements for conducting and the sclerenchyma fibers for mechanical support evolved from tracheids. In fact, the more primitive angiosperms have vessel elements that share morphological features with tracheids. In some conifers, some tracheids may work as storage cells. Some current angiosperm groups, such as winteraceae, have tracheids in the xylem as the only conducting cell type. These tracheids are long cells (4.5 mm) with thin cell walls and blunt ends that overlap with the next and previous tracheid.
Tracheary cells are generated by the proliferation and differentiation of cells from either the procambium meristem or the vascular cambium meristem. In trees, the cell cycle of a vascular cambium cell is long and may last from 10 to 15 days, depending on the species and environment. Once the new cell is produced, it grows by relaxing the cell wall and by the influx of water driven by the inner concentration of substances. In this way, the hydraulic pressure pushes against the cell wall. During cell growth, many molecules need to be synthesized to increase the cell wall surface. These initial processes are crucial, since the cell may increase 10 to 100 times its initial size. Besides water availability, the cell growth is influenced by phytohormones like auxin, gibberellins, and cytokinins. The secondary cell wall is synthesized and formed after the final sized is reached. The secondary cell wall is 2 to 10 µm thick, poorly hydrated, and very stiff. It is the main component of the wood. It is composed of cellulose (40-60 % of the dry weight), hemicellulose (10-40 % of the dry weight), and lignin (10 - 35 % of the dry weight).
Parenchyma cells (c) are another cell type of the xylem. Commonly, the parenchymatic component is more developed in angiosperms than in gymnosperms. It is about 26 % of the xylem in angiosperms and about 6 % in conifers. A higher proportion of parenchyma is found in the xylem of succulents and lianas. In addition, it looks like the higher temperature increases the parenchyma content in angiosperm xylem.
Parenchyma cells are organized in the conducting tissues in two ways: radially or axially, which are perpendicular to one another. Radial parenchyma cells form rows, or rays, perpendicular to the surface of the organ, while axial parenchyma cells form groups, or rows, arranged longitudinally in the xylem and are more abundant in the secondary xylem (see below). The amount and features of axial and radial parenchyma are variable among plant species, and the differences have been used for evolutionary studies. Some plant species lack radial parenchyma.
Radial parenchyma cells are elongated, parallel to the axis of the ray, and connected to one another and to other cells by numerous plasmodesmata that allow the transport of substances. They account for most of the living cells of the xylem. The rays may range from less than one mm to several cm in height and from one to several cells wide. They are named uniseriate when there is only one row of cells, biseriate with two rows, and multiseriate if there are more than two. In some species, several thin rays may join and look like only one, known as aggregated rays. In addition, some plants show only one type of ray while others have different types of rays. Homocellular rays contain parenchyma cells showing a similar cell type, whereas rays showing cells with different features are referred to as heterocellular rays. Heterocelular rays are regarded as ancient compared with homocellular rays. In conifers, the rays are uniseriate or biseriate, whereas in angiosperms, they are usually multiseriate. The rays of the xylem are continuous with the rays of the phloem. This is so because one single cell of the cambium mertistem can differentiate into radial parenchyma cells of either the xylem or phloem. The phloem rays are usually less discerned than the xylem ones.
Axial parenchyma cells are often in contact with conducting cells, with a distribution pattern that depends on the species. However, the axial parenchyma cells are more abundant in the phloem than in the xylem. The axial parenchyma in the xylem is classified as paratracheal when it is associated with the vessel elements and apotracheal when it is not. There are three types of paratracheal parenchyma: vasicentric, when it is in contact with the vessel elements; aliphorm, when it surrounds the vessels and extends away as wings; and confluent, when it forms bands and parenchyma cells surround and contact the vessels. The apotracheal parenchyma can be divided into four types: terminal, when it appears in the last layers of new xylem; limitant, when it appears on both sides of the growing xylem; diffuse, when it is scattered through xylem layers; and banded, when it appears in small bands scattered among the vessels. The apotracheal organization is regarded as primitive compared with paratracheal, which appeared later.
Parenchyma cells perform multiple functions: store carbohydrates (starch), water, nitrogen, and mineral depots, participate in the defense against pathogens, cooperate during lignification of tracheary components, refill those conducting cells that get air bubbles inside (cavitation), influence the mechanical properties of the tracheary components, facilitate communication between xylem and phloem, synthesize metabolites and resins, and so on. For instance, the rays of the xylem can store starch, allow communication between the xylem and phloem, and carry molecules to the vascular cambium, which is very active during some seasons.
Sclerenchyma fibers and sclereids (d) provide support and protection. Some sclerenchyma fibers retain their cytoplasm during a period of their active phase, sometimes very short, whereas others are alive as long as the plant is alive. Sclerenchyma fibers are longer than vessel elements, have a lower diameter, relatively thick cell walls, and pointed ends. In some primitive species, sclerenchyma fibers look like tracheids and are named as fibro-trancheids. Sclerenchyma fibers may show septa that extend from the cell wall and divide the cytoplasm into compartments to store substances, such as starch. The mechanical support function of sclerenchyma fibers and sclereids is mostly performed in angiosperms. In gymnosperms, tracheids carry out both mechanical and conducting functions.
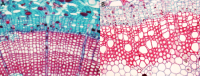
The primary xylem is the first type of xylem developed during the formation of a plant organ. It is protoxylem first, and then metaxylem. The protoxylem is differentiated from the procambium meristem during organ growth. The protoxylem matures completely and disappears later due to the compression of mechanical forces that are produced by the growth of the organ. The cell walls of protoxylem conducting cells, vessel elements and tracheids, show annular thickenings first, and then helical thickenings in cells differentiated later. Because the protoxylem is the first conducting tissue to be functional, it forms near the apical meristems, and therefore it is the main way to supply water and other substances to these meristems. Protoxylem tracheary cells die to be functional but retain the ability to be extended. They assemble a primary cell wall and ring thickenings as the secondary cell wall, which provides mechanical support but does not oppose elongation. These features are also present in cells with helical thickenings in the secondary cell wall. However, the persistent stretching forces during the organ growth lead to the breakage of the cell walls and the cells. The broken cells of the protoxylem are known as protoxylem lacunae. The metaxylem appears after the protoxylem, when the organ enlarges and matures once the growth begins to slow. It also arises from the procambium meristem. Their cells show larger diameters than those of the protoxylem, and the cell walls of the conducting cells have reticulated thickenings first and perforated thickenings later. The metaxylem is the functional mature xylem in those organs that don't go through secondary growth.
The secondary xylem is produced from the vascular cambium meristem in those organs with secondary growth. Together with secondary phloem, it is the mature conducting tissue in plants with secondary growth. The secondary xylem is divided into a radial and an axial component. The radial component consists of cells organized perpendicular to the organ surface, while the axial component, which forms the largest part of the xylem, is organized parallel to the long axis of the organ. The radial component is mostly composed of parenchyma cells, with tracheids in some species. In gymnosperms, the axial component is formed of tracheids and parenchyma cells. Many conifers show resin canals in this tissue. In angiosperms, the axial component consists of tracheids, sclerenchyma fibers, vessel elements, and parenchyma cells. They may also show secretory ducts in this tissue.
The xylem transports The xylem transports water, most of which is intended for transpiration. Only about 5 % of the water transported by the xylem is for cell growth and 1 % for photosynthesis. Hundreds of molecules of water are transpired for each fixed CO2 molecule during photosynthesis. If plants had evolved a mechanism for fetching CO2 without losing water, their need for water would be drastically reduced. On the other hand, transpiration is a saving-energy process for moving water from the roots to the aerial parts until the stomata, because the water is moved by the tension-cohesion properties of water itself.
LLas raíces informan al tallo sobre el entorno del suelo, como la disponibilidad de agua y nutrientes, utilizando el xilema como sistema de comunicación. El xilema también transporta hormonas como el ácido abcísico, el etileno, las citoquinas y otras moléculas desde la raíz hasta las partes aéreas. Además, estas moléculas transportan información sobre la asociación de las raíces con hongos (micorriza) o con bacterias (nódulos) en las leguminosas. Los micronutrientes también son transportados por el xilema. Algunos aminoácidos, como la histidina, la nicotinamida y los ácidos orgánicos se asocian con el hierro, el cobre, el níquel, el zinc y el manganeso. Estos micronutrientes pueden pasar al floema porque también se encuentran allí.
3. Phloem
TThe phloem, also known as sieve tissue or bast, consists of living cells. Its main role is transporting and distributing soluble organic molecules, mostly carbohydrates, synthesized by photosynthesis or mobilized from storing tissues. Besides nutrients, the phloem transports signaling molecules, such as peptides, hormones, proteins, and RNAs. It is a system to ship resources to plant organs. The molecule concentration in phloem is quite high, and the upload with carbohydrates has to be done against the concentration gradient. The phloem is also a pathway for pathogens to produce a systemic infection of the plant. The production of phloem compared with xylem is higher in gymnosperms than in angiosperms.
The phloem is made up of more cell types than the xylem. There are conducting and non-conducting cells. Conducting cells are the sieve cells (a) and sieve tubes (b) (Figures 6, 7, 8, and 9). Both cell types are living cells without a completely functional nucleus, and their primary cell wall is thickened with callose deposits. Only some pinaceae develop a secondary cell wall in the conducting cells. During differentiation, the conducting cells undergo a partial and selective autolysis that removes some organelles. Thus, the vacuole membrane breaks, ribosomes, cytoskeleton, and Golgi apparatus cisterns disappear, and the nucleus is partially degraded. Part of the cytoplasm is conserved with proteins that associate to form fibers, an anastomosed network of smooth endoplasmic reticulum, modified mitochondria, and some plastids. At the cell walls of the cell ends, plasmodesmata are highly modified to form the sieve plates.

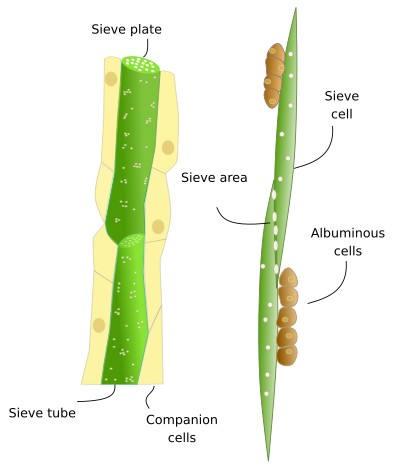
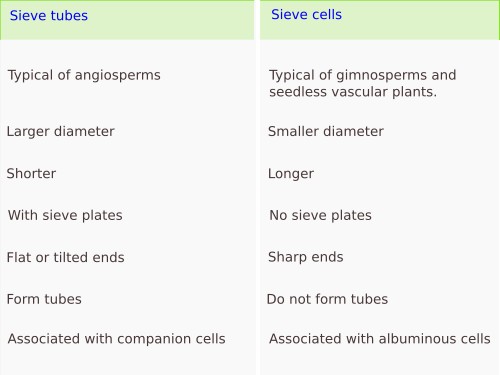
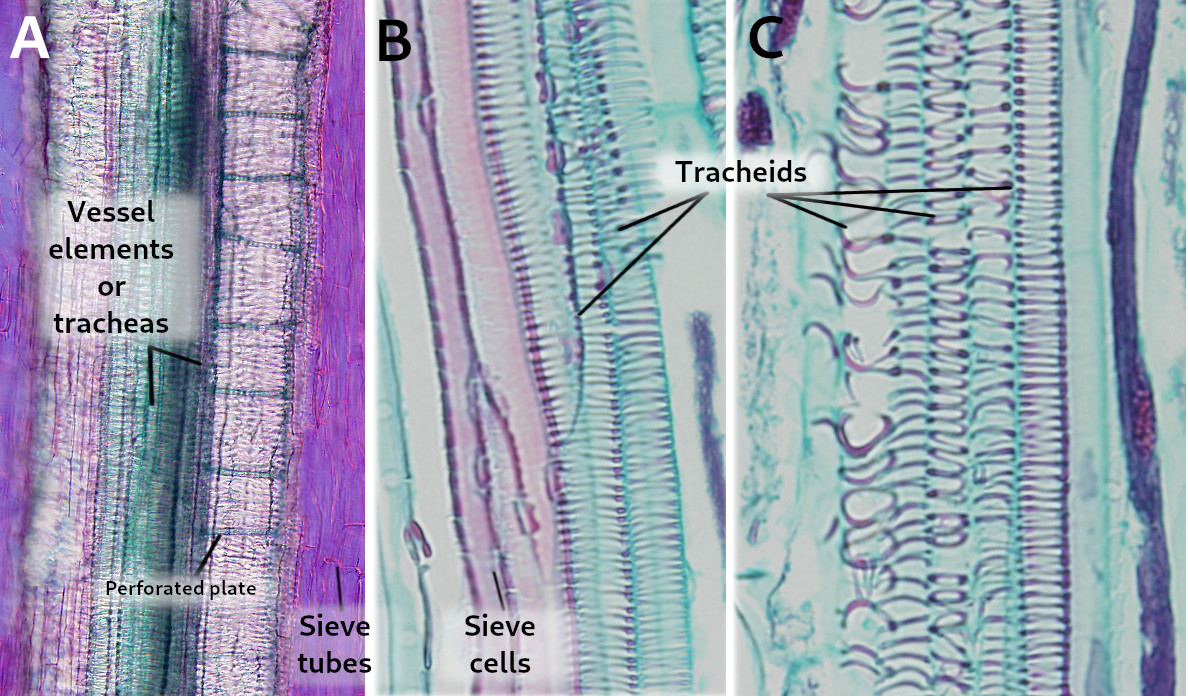
Non-conducting cells are parenchyma cells (c). There is parenchyma associated with sieve tubes and sieve cells. Through asymmetric division, a meristematic cell may differentiate into a conducting cell or an associated parenchyma cell. However, sieve tubes without associated parenchyma cells may also be observed, for instance, in the grass leaves and protophloem of roots. There are also supporting cells (d) in the phloem, such as sclerenchyma fibers and sclereids.
Sieve tubes (a) (Figures 6, 7, 8, and 10) are the typical conducting cells of angiosperms. These are among the most differentiated cells of the plant. Sieve tubes are cells lined up into longitudinal rows that communicate with each other through sieve plates located at both ends of each cell. Sieve plates have large-size pores that allow the direct connection between adjoining cytoplasms. They are formed by the coalescence of fields of pits. This can be compound sieve plates when they are formed from many pit areas, or sieve fields, in more primitive plants, or simple sieve plates when they are formed from a very large sieve field in more modern species. In addition, there are sieve fields with plasmodesmata on the side walls of sieve tubes, with a pore diameter that enlarges as the tissue gets older. The lateral sieve fields communicate adjacent sieve tubes and sieve tubes with parenchyma cells, known as companion cells. In angiosperms, many sieve tubes have pores with callose depots. However, it is not known if these cylinder-like callose depots are functional in living cells since they are present in those damaged or non-functional sieve tubes. Occasionally, callose depots can cover the entire sieve area.
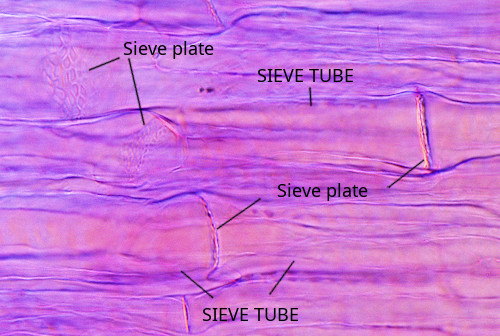
The development of sieve plates takes place during the sieve tube differentiation. There are three phases: enucleation, thickening of the cell wall, and formation of the sieve plate. Initially, only the middle lamella of the cell wall separates the two daughter cells. Callose depots are laid on both sides of the middle lamella. Between two neighboring callose depots, the primary cell wall is assembled. Finally, the callose depots get thinner, and the middle lamella is degraded, forming a channel that communicates both cytoplasms. The pore may remain surrounded by a ring of callose or completely clean.
Sieve cells (b) (Figures 7, 8, 9, and 10) are typical of gymnosperms, pteridophytes, and other plants with primitive features. They are long cells with pointed ends, communicating with each other laterally by primary pore fields forming the sieve areas. They don't have sieve plates. Functionally and morphologically, sieve cells are associated with a type of specialized parenchymal cell called Strassburger's cells (albuminous cells). Sieve cells are the only conductive element in gymnosperms and pteridophytes. Like sieve tubes, sieve cells lose most of their cytoplasmic content during differentiation. In some pinaceae may be sieve cells with a secondary cell wall.

Parenchyma cells (c) are components of the phloem. Companion cells are parenchyma cells strongly associated with the sieve tubes of angiosperms. This communication is mediated by plasmodesmata. They maintain the metabolism of sieve tubes. In fact, the functional unit of the angiosperm phloem is the sieve tube/companion cell. Commonly, a companion cell is associated with only one sieve tube. Both cell types are differentiated from the same meristematic cell. The companion cells maintain sieve tubes alive, as well as upload and download the transported organic substances. Companion cells contain a large nucleus and a cytoplasm full of organelles, which means a high metabolic rate. However, they do not store starch. The cytoplasm of companion cells is usually darker than sieve tubes. In stems, sieve tubes and companion cells are initially connected with other phloem cells, but plasmodesmata are progressively closed, and only those connecting sieve cells with companion cells remain.The size of companion cells is relatively larger when compared with sieve tubes in the uploading organs, such as leaves and storing organs. In the mesophyll of leaves, the companion cells are involved in the uploading of sieve tubes with photosynthesized compounds through pores connected to the sieve fields. However, both cell types are relatively isolated from the surrounding cells in the stem. In the downloading organs, the companion cells are scarce, and the sieve tubes are connected with other neighboring parenchyma cells.
In gymnosperms, the parenchyma cells associated with the sieve cells are known as Strasburger cells, or albuminous cells (c) (Figure 6). Although they have similar functions, the origin of Strasburger cells is different from that of companion cells of angiosperms. They are marginal cells in contact with the sieve cells and show a cytoplasm with many organelles. Strasburger cells are connected to sieve cells through sieve fields/plasmodesmata complexes and with radial parenchyma cells through plasmodesmata
Other types of parenchyma cells (c) are present in the phloem. Some of them work as storage for substances transported by the phloem itself. In some species, there are cells in the phloem specialized for secretion. In conifers, large axial parenchyma cells are observed in the secondary phloem.
Sclerenchyma fibers and sclereids (d) are found in the phloem with supporting and protection roles. Sclerenchyma fibers are abundant in the secondary phloem. They are large and pointed cells, often showing thick secondary cell walls.
The primary phloem is the first phloem to be functional in developing organs. The first type of primary phloem to appear is the protophloem, which is differentiated from the procambium meristem. In angiosperms, protophloem shows non-well-developed sieve tubes, while in gymnosperms and ferns, it shows poorly-developed sieve cells. Companion cells are rare, absent, or are generated once the protophloem is differentiated. The protophloem is generated before the protoxylem and, therefore, is closer to the apical meristem. This allows a supply of nutrients to the apical meristems. If the protophloem is not fully functional, the apical meristem is not organized properly. Metaphloem replaces protophloem during development, usually when the organ stops growing. It is also differentiated from the procambium meristem. The metaphloem contains sieve tubes, or sieve cells, that are thicker and longer than in the protophloem. In angiosperms, it always contains companion cells, and Strasbuger cells in gymnosperms. The sieve tubes have sieve plates. Metaphloem is the functional conducting tissue in plants and organs with primary growth.
Morphologically, it is difficult to distinguish the protophloem from metaphloem. They are named according to the time they appear during organ developement. Protophloem is present in elongating parts, while metaphloem in those regions where primary growth is stopped. In protophloem, the sieve tubes are usually associated with parenchyma cells that later become sclerenchyma fibers, which will form the sclerenchyma sheath in the vascular bundles. The companion cells are observed in the metaphloem. In the primary phloem, there are fibers arranged in cords intended to protect the phloem from being crushed by the other tissues. However, the primary phloem is compressed during the secondary growth and stops being functional.
The secondary phloem arises from the vascular cambium meristem in plants with secondary growth. The conducting cells and associated parenchyma in the secondary phloem are similar to those of the primary phloem. The conducting cells are well-developed, as are the companion cells, and both axial and radial parenchyma are present. The axial parenchyma cells may be difficult to differentiate from the associated parenchyma. Unlike in xylem, secondary phloem cells do not synthesize secondary cell walls, and therefore they are living cells. In the trees, the secondary phloem involved in conducting nutrients is scarce, and the sieve tubes live for about a year because they are crushed by the growing of the secondary xylem.
In plants, the sap flux through the phloem conducting cells is powered by concentration gradients. This model of fluid propelling was suggested by Münch at the beginning of the 20th century. The gradients are enough to boost the conduction even in the tallest trees, although the speed of the sap in these trees may be 10 times lower than in herbaceous plants
The substances transported through the phloem are vital for plants. transport is a three-step process: upload of conducting cells of source organs, mostly leaves; transport through the sieve tubes (or sieve cells); and download of the substances in the target tissues. The osmotic gradient is the power that boosts the sap along the phloem. As mentioned before, many types of organic molecules are driven through the phloem, such as proteins, RNAs, carbohydrates, and signaling molecules like hormones. In the leaves, these molecules are uploaded in three ways: apoplastic, active symplastic, and passive symplastic (Figure 12). It is unknown why one of these methods is selected in a particular plant species. In the apoplastic method, the carbonated substances are synthesized in the cells of the leaf mesophyll and reach the cytoplasm of the phloem parenchyma cells by diffusion. Then the transported molecules are released into the extracellular space (apoplastic) by plasma membrane transporters. The companion cells (in angiosperms) uptake the molecules through plasma membrane transporters, and once inside the cells, the molecules arrive in the sieve tubes by diffusion. In the active simplastic way, carbohydrates get to the companion cells through plasmodesmata. Then carbohydrates are transformed into oligomers, preventing them from crossing plasmodesmata again, but not the diffusion toward the sieve tubes because the pores communicating companion cells and sieve tubes are larger. In the passive simplastic method, carbonated molecules arrive in the sieve tubes by concentration gradient. Aquaporins are water membrane channels that facilitate these osmotic pressures. The three ways of uploading the phloem may coexist in the same plant or alternate between them, depending on the circumstances. Specialized companion cells are needed for each uploading method.
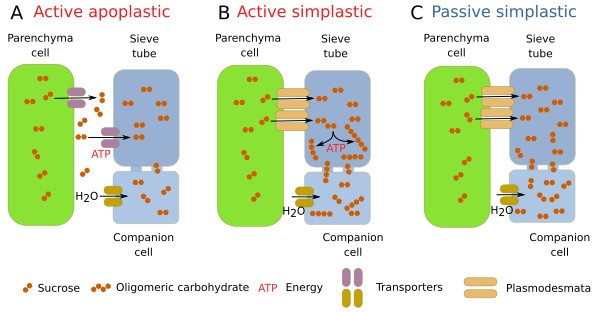
During the daytime, the phloem is uploaded with carbohydrates produced during photosynthesis, and during the nighttime, the uploaded substances come from starch depots and vacuoles. In addition, the conduction along the phloem, which is relatively fast, is not much affected by transpiration. The speed of conduction depends on the size of the sieve tubes and the size of the pores on the sieve plates. It is thought that the potential conduction capacity of phloem is high, and it is more influenced by the download process in the target organs. The power for conduction is generated by osmosis at the upload regions. In the downloading regions, the output flux is greatly influenced by plasmodesmata communicating sieve tubes and parenchyma cells.
IIn the target organs, the molecules transported by the phloem leave sieve cells by passive facilitated diffusion or by carbohydrate transporters, for instance, by carbohydrate/proton simport. In any case, the phloem loses water, since water goes along with organic molecules during downloading. The phloem has to deliver many molecules in many places, and the amount of molecules depends on the demands and state of each target region.
-
Bibliography ↷
-
Furuta KM, Hellmann E, Helariutta Y. 2014. Molecular control of cell specification and cell differentiation during procambial development. Annual review of plant biology. 65:607-638.
Lalonde S, Francesch VR, Frommer WB. 2001. Commpanion cells. In: eLS. John Wiley & Sons Ltd, Chichester. http://www.els.net [doi: 10.1038/npg.els.0002087]
Liesche J, Patrick J. 2017. An update on phloem transport: a simple bulk flow under complex regulation. F1000Research 6(F1000 Faculty Rev): 2096
.
Lucas WL, Groover A, Lichtenberger R, Furuta K, Yadav S-R, Helariutta Y, He X-Q, Fukuda H, Kang J, Brady SM, Patrick JW, Sperry J, Yoshida A, López-Millán A-F, Grusak MA, Kachroo P. 2013. The Plant Vascular System: Evolution, Development and Functions. Journal of integrative plant biology. 55: 294–388.
Scarpella E, Meijer AH. 2004. Pattern formation in the vascular system of monocot and dicot plant species. New phytologist. 164: 209-242.
Spicer R. 2014. Symplasmic network in secondary vascular tissues: parenchyma distribution and activity supporting long-distant transport. Journal of experimental botany. 65: 1829-1848.
-