1. Morphology
2. Protein synthesis
- Quality control
3. Lipid synthesis
4. Detoxification
5. Glucose homeostasis
6. Calcium store
7. Vesicles
8. Membrane contact sites
9. Peroxisomes
10. Lipid droplet
11. Autophagy
The endoplasmic reticulum is an intricate membrane-bound compartment arranged in interconnected tubules and flattened cisterns. It is divided into three domains: the rough endoplasmic reticulum, smooth endoplasmic reticulum and the nuclear envelope. The rough endoplasmic reticulum tubules and flattened cisterns show associated ribosomes with their membranes (that is why the name rough). The smooth endoplasmic reticulum is organized in irregular and convoluted tubules, with no associated ribosomes. The name reticulum was given after the first observations at transmission electron microscopy because of its network-like organization. The nuclear envelope may be regarded as a third domain because it is physically continuous with the membranes of the endoplasmic reticulum. Although the nuclear envelope performs specific functions, ribosomes associated with the outer membrane of the nuclear envelope are observed. The main functions of the endoplasmic reticulum are the synthesis of proteins, lipids and glycoconjugates, calcium storage, and nuclear organization. However, there are many other functions performed by this organelle. The endoplamic reticulum is the largest organelle in most animal cells.
Despite its size, the endoplasmic reticulum was described at light microscopy quite late compared with other cell organelles. It was first described by A. Veratti (a disciple of Camilo Golgi) in 1902. In the 1950s of the 20th century, it was observed by V. Porter and G. Palade at electron microscopy. Porter gave it the name reticulum because of its intricate nature.
1. Morphology
The endoplasmic reticulum is a complex system of membranes organized in tubules and flattened cisterns connected to each other and sharing the same luminal space. The endoplasmic reticulum membranes can be found all through the cytoplasm, from the nuclear envelope to near the plasma membrane. They may account for more than half of the total membranes of a typical animal cell, and they can be about 10 times larger than the plasma membrane.
This organelle is divided into three main functional and morphological domains. The rough endoplasmic reticulum has ribosomes associated with its membranes. The smooth endoplasmic reticulum does not show associated ribosomes (Figures 1 and 2). The nuclear envelope is the third domain because its membranes are continuous with the cytoplasm endoplasmic reticular membranes. We will deal with the nuclear envelope in the pages about the cell nucleus (see nuclear envelope). In plant cells, there is another functional domain of the endoplasmic reticulum found in the plasmodesmata: the desmotubule.
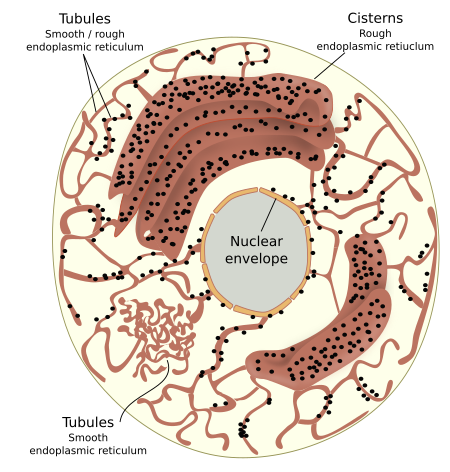

Rough and smooth endoplasmic reticulum may not share the same cytoplasm region. This non-overlapping distribution is observed in hepatocytes, neurons and cells synthesizing steroid hormones. However, in some regions, there is no clear separation between both domains, and tubules with associated ribosomes are intermingled with naked tubules. The spatial distribution of the endoplasmic reticulum through the cytoplasm is set by interactions with the cytoskeleton, mostly microtubules in animal cells and actin filaments in plant cells. In animal cells, the formation of new tubules depends on the traction forces generated by motor proteins and microtubules. Thus, endoplasmic reticulum membranes are literally dragged over the microtubules resulting in a new tubule. Furthermore, it has been observed that a tubule may contact the plus end of a microtubule so that the polymerization of the microtubule pulls and extends the length of the tubule. However, the cytoskeleton does not seams to influence the shape of the endoplasmic reticulum, tubules or cisterns, once they are formed
How the endoplasmic reticulum is organized in the cytoplasm is influenced by the cell type and the physiological state. Tubules and cisterns are continuously interconverted in the cytoplasm, and the reticular arrangement is always under remodeling. It often happens in hepatocytes, which perform a large variety of metabolic processes, and this is reflected in the endoplasmic reticulum organization. Besides, cisterns disappear during mitosis, and nearly all the endoplasmic reticulum is arranged as tubules. Those cells specialized in one function usually show a long-lasting endoplasmic reticulum organization. For instance, secretory cells maintain a high proportion of cisterns, and those dedicated to lipid synthesis show many smooth endoplasmic reticulum tubules.
As mentioned above, cisterns are more abundant in secretory cells (Figure 3), where cistern stacks are observed. Adjoining cisterns are connected by membrane bridges that allow them to share the same luminal space. More secretory activity means more cisterns and more tightly piled. For instance, in the secretory cells of salivary glands, the distance between neighboring cisterns is about 84 nm, whereas it is about 270 nm in neurons. Cisterns are specialized in protein synthesis and folding. It has been suggested that the cistern morphology is better than tubules to perform these functions more efficiently because there are large and flat membrane regions where many ribosomes, in polysome form, can be accommodated, as well as translocons and other proteins involved in translation (see below). Moreover, the volume/surface ratio of cisterns is higher, so protein folding and quality control have more space.

Tubules form a polygonal network that is always changing by breakages and fusions. They are much more dynamic than cisterns. It is interesting that the diameter of tubules and the thickness of cisterns are similar in cells of the same species. For instance, it is about 30 nm in yeasts and 50 nm in mammals. It is also interesting that the separation of the inner and outer membranes of the nuclear envelope is 50 nm in mammals. In cisterns, the transmembrane protein Climp-63 localizes in the opposite membranes of the same cistern, and the interaction of their luminal domain determines the membrane separation. Its cytosolic domain can associate with microtubules, and the increase in the expression of Climp-63 leads to the conversion of tubules into cisterns. Other proteins, such as RRBP1, collaborate to get the flattened morphology of cisterns. The number of ribosomes doing translation associated with the endoplasmic reticulum membranes also affects the flatten morphology. When the number of associated ribosomes increases, the tubule tends to flatten. The density of ribosomes in cisterns may be up to 1000 per µm2, whereas it is much lower in tubules. Although tubules harbor all the protein translation machinery, they cannot make room for large polysomes or a high density of ribosomes.
Tubule organization is influenced by the reticulon and DP1 proteins. Both show a wedge-like 3D conformation that bends the membranes where they are inserted. In humans, there are 7 genes for reticulons that may lead to 11 isoforms. Once inserted in the endoplasmic reticulum membranes, these proteins expand the cytosolic hemilayer compared to the luminal hemilayer, leading to a membrane bend. These proteins stabilize the tubule organization. Reticulon and REEPS proteins (Yop1p in yeasts) transform tubules into cisterns, and the opposite happens when they are not present. There are other proteins affecting the membrane curvature, such as ARL6IP1, espastin and FAM134. Furthermore, the cytosolic domains of all these proteins interact with other cytoplasmic elements, such as mitochondria, vesicles, the cytoskeleton, and even the plasma membrane.
Tubules also bear atlastin GTPase, lunapark, Rab 10 and Rab 18, which drive the fusion of tubules to form a network and make possible tripartite junctions (connection of three tubules). Atlastins are related to dynamines and show a similar structure than mitofusins, which are involved in mitochondrial fusion. However, the general organization of the net of tubules is generated and maintained by the cytoskeleton. Other elements participate in the tubule network dynamics. For instance, the fatty acid chains of lipids in the endoplasmic reticulum membranes are shorter than those of other membranes, and therefore the endoplasmic reticulum membranes are more fluid.
2. Protein synthesis
Protein synthesis is the main function of the rough endoplasmic reticulum. This activity is intense in secretory cells and necessary for every cell. It is estimated that about 1/3 of the total proteins in a cell are synthesized in the endoplasmic reticulum. The proteins end up in different places: a) the extracellular space; b) the lumen of organelles involved in the vesicular trafficking; c) as integral components of membranes, both the plasma membrane and the membrane of cell organelles participating in the vesicular trafficking; and d) as resident proteins. For instance, all transmembrane proteins of the plasma membrane are synthesized in the endoplasmic reticulum. The endoplasmic reticulum needs to synthesize proteins for itself, which are known as resident or constituent proteins. The shipment of proteins to their correct destination depends on particular amino acid sequences, or molecular motifs, that are recognized by the sorting mechanisms.
All these proteins begin to be synthesized by free ribosomes in the cytosol, and the synthesis ends with the protein either free in the lumen or as part of the reticulum membrane (Figure 4). The process of protein synthesis starts when an mRNA joins a small ribosomal subunit, and then a large ribosomal subunit, to begin with translation. The first translated segment of the mRNA is a sequence of amino acids known as the signal peptide, which is about 70 amino acids long. There is not a consensus sequence for all these proteins, but it is characterized by having about 30 hydrophobic amino acids, a short polar tail, and a short basic sequence. This sequence is recognized by a cytosolic molecule called SRP (sequence recognition particle), and then the translation process slows down. SRP contains a molecular cavity with methionine amino acids, where the hydrophobic sequence of the signal peptide docks. Then, SRP interacts with the ribosome by binding to the elongation factor, which is necessary for translation. Therefore, the SRP bond to the signal peptide stops translation. At the same time, SRP exposes a hidden amino acid sequence that is later recognized by a receptor found in the endoplasmic reticulum membranes.

The mRNA-ribosome-SRP-signal-peptide complex diffuses through the cytosol until it hits an endoplasmic reticulum membrane. In these membranes, there are SRP-receptors for recognizing the bonded SRP. The whole complex becomes attached to the membrane and then interacts with a multiprotein complex called translocon. The translocon is made up of enzymes and other proteins, as well as three transmembrane proteins that form a hydrophilic channel. This channel is open when there is an ongoing translation, but closed when there is not. This is a necessary feature because the endoplasmic reticulum is a calcium store, and calcium ions can diffuse through the hydrophilic channel. After association with the translocon, SRP and SRP-receptor are released, and the mRNA-ribosome-signal-peptide complex is attached to the translocon. Then, the translation is resumed, the signal peptide is attached to the channel, and the nascent polypeptide grows inside the translocon channel. If the final protein is soluble in the lumen, a peptidase found in the endoplasmic reticulum cleaves the signal peptide, while the rest of the protein falls into the lumen of the endoplasmic reticulum. The nascent polypeptide is quickly folded by chaperones to get a proper spatial conformation. When translation is finished, the ribosome and the mRNA are disengaged from the translocon, and they are free for another round.
Transmembrane proteins are not released into the lumen of the endoplasmic reticulum but are inserted into its membrane. Transmembrane proteins contain sequences of hydrophobic amino acids. When these sequences are being translated and going through the translocon channel, they can cross the wall of the translocon and get among the fatty acid chains of membrane lipids. The process is rather complex and diverse, depending on the protein. For instance, some receptors are transmembrane proteins with a chain of amino acids that can cross the cell membrane seven times, alternating transmembrane amino acid sequences with hydrophilic sequences that have to be placed in the lumen or in the cytosol. Although it is not common in animal cells, the endoplasmic reticulum may import some proteins completely synthesized in the cytosol thanks to other proteins associated with its membrane.
It is also worthy to mention that the endoplasmic reticulum membranes facilitate the synthesizing activity because they are thinner (about 5 nm) and more fluid, so that the insertion and folding of peptides are facilitates. A higher proportion of glicerophospholipds is present with a low rate of saturation (less than 15%), and there is no net electrical charge on the cytosolic surface.
Proteins in the rough endoplasmic reticulum are modified while they are being synthesized. a) Asparagine amino acids are glycosysilated (N-glycosylation) with a molecular complex composed of 14 monosaccharides. Each of these trees of carbohydrates is first assembled in a molecule of dolichol phosphate, a membrane lipid, and then transferred to an asparagine of the nascent protein. In the Golgi apparatus, some terminal monosaccharides of these complexes will be lost, and other saccharides will be added. b) Some proteins, mostly those targeted to the extracellular matrix, are hydroxylated. In this way, proline and lysine amino acids of the collagen molecules end up as hydroxyproline and hydroxylysine. c) Some proteins of the plasma membrane are chemically bonded to some membrane lipids. This chemical bond is also established in the rough endoplasmic reticulum. d) Disulfide bonds between polypeptide chains are formed in the endoplasmic reticulum.
Protein quality control. ERAD.
Proteins may fail to fold properly for many reasons. A misfolded protein may be dangerous for the cell. Failures in the folding process happen more often than one might expect. In fact, protein folding is the point most likely to fail in the sequence of gene expression, that is, from transcription to the functional protein. For instance, the CFRT protein (cystic fibrosis transmembrane regulator) has an 80 % failure rate during protein folding, compared with other stages of the gene expression process..
A quality control mechanism for synthesized proteins is working in the rough endoplasmic reticulum so that defective proteins are removed from the organelle and degraded in the cytosol. Only proteins with a proper spatial conformation are allowed to enter the secretory pathway. Chaperones are proteins that help newly synthesized proteins to achieve the correct spatial conformation. They also play an essential role in detecting defective proteins and targeting them for degradation. Other proteins bearing lectin domains are able to recognize a wrong arrangement of carbohydrates. The molecular mechanism for degrading malformed proteins is called ERAD (ER associated degradation).
The ERAD system for soluble proteins begins in the endoplasmic reticulum lumen with chaperones recognizing a misfolded protein and follows with the translocation of this protein across the organelle membrane, the ubiquitination of the protein in the cytosol, modification by a AAA-ATPase and degradation by proteosomes (Figure 5). For misfolded transmembrane proteins, the process is similar, but they may be ubiquitinated first in its cytosolic domain and then extruded from the membrane. The ERAD system is actually a complex network with molecular pathways running at the same time.

The ERAD mechanism performs other functions, such as lipid homeostasis, maintaining the endoplasmic reticulum identity, organizing the nuclear envelope, it is involved in membrane contact sites, calcium flux, and signaling in the innate immune system.
When misfolded proteins increase in number and the ERAD system is not enough to remove them, they accumulate in the endoplasmic reticulum. Misfolded proteins may amass for many reasons: increase in protein synthesis, flaws in the ubiquitin-proteosome degradation, defective autophagy, shortcoming of energy, low level of nutrients, calcium concentration dysregulation, failure of the oxido-reduction balance, hypoxia, and so on. To remove the misfolded proteins, several molecular pathways are activated in the endoplasmic reticulum that restore proteostasis (protein homeostasis) or initiate cell death by apoptosis if the problem cannot be solved. These molecular pathways are collectively known as the unfolded protein response (UPR) and lead to an increase in the expression of genes for new chaperones to fix the misfolded proteins, slow down the synthesis of new proteins, as well as increase protein degradation. The URP may even trigger the degradation of the endoplasmic reticulum by autophagy.
In Parkinson and Alzheimer neurodegenerative diseases, there are mutations leading to overproduction and aggregation of misfolded proteins, such as beta-amyloid and alpha-syncluclein, respectively. The UPR system tries first to prevent the accumulation of these proteins. However, the production of misfolded proteins is so heavy that proteostasis cannot be recovered, and the apoptotic pathway is initiated, which leads to cell death. The UPR seems to be involved in some metabolic diseases related to glucose and lipid regulation, as well as cancer.
In plant cells, protein synthesis may be intended for storing energy. These proteins are targeted to vacuoles, although some plant species can also store these proteins in the endoplasmic reticulum.
3. Lipid synthesis
Most membrane lipids are synthesized in the smooth endoplasmic reticulum, including glycerophospholipids and cholesterol. Although a large part of the sphingolipid molecules are assembled in the Golgi apparatus, their basic component ceramide is synthesized in the endoplasmic reticulum. The smooth endoplasmic reticulum is actually a platform for assembling membrane lipids and not a center for the synthesis of lipids from scratch. Thus, fatty acids synthesized in the cytosol, or coming from food, are inserted in the cytosolic hemilayer of the endoplasmic reticulum membrane, and then other molecules are sequentially added to form glycerophospholipids. The assembling process is carried out by enzymes with their activi centers facing the cytosol. Hence, all lipids are initially formed in the cytosolic hemilayer. Since flip-flop movements are nearly forbidden for lipids because of the hydrophobic environment of fatty acid chains, they need help to be transferred to the luminal hemilayer (that facing the lumen). There are specialized proteins that can move lipids from one hemilayer to the other: flippases, floppases and scramblases. Although the endoplasmic reticulum membrane has slightly more phosphatidyl serine and less phosphatidyl inositol in the luminal hemilayer than in the cytosolic hemilayer, both hemilayers show similar composition, and it is therefore symmetric. Thus, the membrane asymmetry present in other organelles is due to the differential work of flipases, flopases and translocases, and to specific metabolic processes that remove lipids or transform a lipid type into another one. Thus, the final place of a lipid does not really depends on its synthesis in the endoplasmic reticulum membranes.
Cholesterol is another mayor lipid for the plasma membrane and other post-Golgi membranes that is synthesized in the endoplasmic reticulum. It is transported by vesicular trafficking and by carriers. For instance, yeast move ergosterol (they do not have cholesterol) from the endoplasmic reticulum to the plasma membrane by a pathway that circumvents vesicular trafficking. There are a variety of cholesterol carriers, and this transport does not need ATP.
Vesicles transport the bulk of lipids as components of their own membranes between cell organelles (Figure 6). Mitochondria, chloroplasts and peroxisomes are not major players in vesicular trafficking, so some lipids have to be imported by other means. For example, glycerophospholipids are transported by a cytosolic protein known as the glycerophospholipid interchanger. This carrier can take a lipid from the membrane of the smooth endoplasmic reticulum, travel with it through the cytosol, and leave it in the membrane of another organelle. Another way to transfer lipids between organelles occurs at the membrane contact sites. At electron microscopy, it has been observed that membranes of the endoplasmic reticulum and other organelles, such as mitochondria and peroxisomes, are in close apposition. Lipids are transferred between the two membranes mediated by a carrier at these membrane contact sites. On the other hand, new peroxisomes are formed with membranes coming from the endoplasmic reticulum and mitochondria. Mitochondria and chloroplasts are able to locally synthesize some lipids for their membranes. Thus, chloroplasts synthesize glycerolypids and glycolipids, although some lipids are transferred at the endoplasmic reticulum-chloroplast membrane contact sites.

Triacylglycerols are also synthesized in the smooth endoplasmic reticulum. These lipids are stored in the reticulum itself or as lipid droplets. The synthesis of triacylglycerols is heavy in adipocytes, the fat-storing cells of animals that shows large lipid dropolets. This fat-storing organelle is an energy source for the body and, in some species, for thermal insulation as well. Lipids synthesized in the smooth endoplasmic reticulum are also needed for lipoproteins, steroid hormones and bile acids. For instance, the Leydig cells in the testis synthesize steroid hormones and show a well-developed smooth endoplasmic reticulum.
4. Detoxification
Hepatocytes, the liver cells, show a highly developed smooth endoplasmic reticulum. Lipoproteins that transport cholesterol and other lipids to other parts of the organism are synthesized here. In the smooth endoplasmic reticulum membranes, the P450 protein family is in charge of removing potentially toxic metabolites as well as liposoluble toxins incorporated during digestion. The surface of the smooth endoplasmic reticulum is adapted to the amount of detoxifying enzymes, which in turn depends on the amount of toxic substances. The tubules shape and the lack of ribosomes expose a large surface of membrane compared to the organelle luminal volume.
5. Glucose homeostasis
Glucose is usually stored as glycogen, primarily in the liver. This organ is the main source of blood glucose. Two hormones regulate the glucose release from the liver into the blood: insulin and glucagon. The catabolism of glycogen yields 6-phosphate glucose, which cannot cross the plasma membrane and hence cannot leave the cell. Glucose 6-phosphatase, which is anchored to the endoplasmic reticulum, removes the phosphate residue, allowing glucose to be moved out of the cell.
6. Calcium storage
The endoplasmic reticulum is specialized in capturing and storing cytosolic calcium by means of calcium pumps found in its membrane. The calcium concentration in the endoplasmic reticulum lumen is up to milimolar, while in the cytosol it is nanomolar. The stored calcium may massively get out of the endoplasmic reticulum after intracellular or extracellular cues and then trigger cellular responses, such as exocytosis. The sarcoplasmic reticulum (the muscle cell endoplasmic reticulum) controls the contraction and relaxation of muscle cells by alternating between calcium uptake and calcium release.
7. Formation of vesicles
See this page.
8. Membrane contact sites
At electron microscopy, membranes of the endoplasmic reticulum are often observed in close apposition to membranes of other organelles, such as the Golgi apparatus, lipid droplets, and plasma membrane.
Endoplasmic reticulum-plasma membrane. In yeasts, about 20 % to 40 % of the inner surface of the plasma membrane is coated with endoplasmic reticulum membrane. There are at least six proteins involved in maintaining this close position of both types of membranes, and some of them have the ability to transfer lipids between membranes. Thus, it is thought that lipid exchange happens at these membrane contact sites. In mammals, the contact sites between the endoplasmic reticulum and the plasma membrane are also frequent. However, their main function is the transmission of an electrochemical gradient.
Endoplasmic reticulum-mitocondria. Membrane contact sites between the endoplasmic reticulum and mitochondria are easily observed. The levels of intracellular calcium seem to be modulated by these contacts because both are calcium storage organelles. In addition, lipid exchange happens between both membranes, mainly transferring phospholipids from the endoplasmic reticulum to mitochondria. Another function that has been backed to these membrane contact sites is the fission of mitochondria because proteins needed for mitochondrial division are recruited to these spots. In fact, the mitochondrial fission begins with a ring of endoplasmic reticulum membranes wrapping a mitochondria, and later the fission of mitochondrial membranes in this region. It has also been suggested that these membrane contact sites are involved in the formation of autophagosomes.
Endoplasmic reticulum-Golgi apparatus. Endoplasmic reticulum membranes are often found in contact with membranes of the trans cisterns of the Golgi apparatus, where ceramide and sterols are transferred from the endoplasmic reticulum to the Golgi apparatus. Ceramide are needed for sphingolipid synthesis, which happens in the Golgi apparatus.
Endoplasmic reticulum-endosomes. The endoplasmic reticulum seems to affect some aspects of the life of endosomes, such as division by recruiting necessary proteins, maturation and movement by modifying molecules that interact with the cytoskeleton. As in mitochondria, rings of endoplasmic reticulum membranes have been observed wrapping endosomes, so it looks like these are structures for endosome division. Furthermore, it has been suggested that cholesterol is transferred from the endoplasmic reticulum to endosome membranes.
9. Peroxisome formation
See this page.
10. Lipid droplet formation
See this page.
11. Autophagy
See this page.
-
Bibliography ↷
-
Bibliography
Arruda AP, Parlakgül G. 2022. Endoplasmic reticulum architecture and inter-organelle communication in metabolic health and disease. Cold spring harbor perspectives in biology. 15(2):a041261. doi: 10.1101/cshperspect.a041261.
Christianson JC, Carravalho P. 2022. Order through destruction: how ER-associated protein degradation contributes to organelle homeostasis. The EMBO journal. 41: e109435. DOI 10.15252/embj.2021109845.
English AR, Zurek N, Voeltz GK. 2009. Peripheral ER structure and function. Current opinion in cell biology. 21:506-602.
Daleke DL. 2007. Phospholipid Flippases. The journal of biological chemistry. 282:821-825.
Nixon-Abell J, Obara, CJ, Weig VA, Li D, Legant WR, Xu CS, Pasolli HA, Harvey K, Hess HF , Betzig E, Blackstone C, Lippincott-Schwartz3 J. 2016. Increased spatiotemporal resolution reveals highly dynamic dense tubular matrices in the peripheral ER. Science. 354: 3928-2.
-