The golgi apparatus was discovered by Camillo Golgi in 1889, when he was studying neurons. However, it was no accepted as a true organelle for decades. The peryodic acid-Schiff reaction stain carbohydrates and makes possible to visualize the Golgi apparatus at light microscopy. However, the membrane organization of the Golgi apparatus was described in detail by Dalto and Felix (1954) by using transmission electron microscopy. They proposed the name Golgi apparatus.
1. Morphology
In animal cells, the Golgi apparatus is commonly found close to the centrosome, which in turn it is commonly found near the nucleus. This position is set by the interactions of Golgi membranes with microtubules. Microtubules are radially oriented with their minus ends anchored to the centrosome. The Golgi apparatus is made up of flat cisterns piled in stacks. Each stack is referred to as dictyosome (Figures 1). Cisterns are generally thinner in the middle and thicker in the edges (like pizzas), and are more or less bent, showing a convex and a concave surface. Several stacks are present in one cell, and some cisterns connect laterally adjoining stacks (Figure 2). The number (normally 3 to 8) and the size of the cisterns in one stack are variable and depend on the cell type, as well as on the cellular physiological state. A typical cistern is 50 nm thick and it is separated about 15 nm from neighboring cisterns. In one cell, the Golgi apparatus includes all the stacks and their lateral connections.

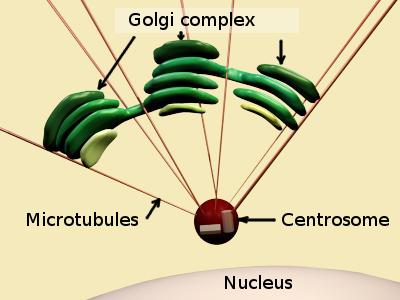
In animal cells, the Golgi apparatus organization is set by several molecular complexes. There is a fibrous protein matrix among the Golgi cisterns that helps to maintain the Golgi apparatus structure. GRASP (Golgi ReAssembly Stacking Proteins) are components of this matrix. They are associated to the cytosolic face of the Golgi cistern membranes. GRASP associated to neighboring cistern membranes may interact between each other, working as glue, to keep the cisterns united in piles. Among the cisterns, there are microtubules and actin filaments, proteins that also form the matrix of the Golgi apparatus. Overall, the matrix proteins are responsible for the Golgi apparatus morphology and for keeping it close to the centrosome.
It has been shown that the structural integrity of the Golgi apparatus mainly relies on the microtubule organization. For instance, the typical cistern stacks organization observed during interphase disappears during mitosis. During mitosis, the Golgi cisterns are split into small vesicles scattered through the cytoplasm, and thereby present in the cytoplasm of the two daughter cells. Once cell division is accomplished, vesicles reunited to form cisterns, and cisterns associted near the centrosome to form the stacks. The position of the Golgi apparatus depends on microtubules nucleated from the centrosome (Figure 3), whereas the stack integrity is maintained by microtubules nucleated from the Golgi cisterns themselves. Microtubules nucleate from gamma-tubulin rings associated to the cistern membranes. Actin filaments and myosin motor proteins help to fine-tune the final location of Golgi stacks. In addition, the Golgi apparatus structure depends on the vesicular trafficking between the Golgi apparatus and the endoplasmic reticulum. Should this trafficking stop, the Golgi apparatus would disappear.
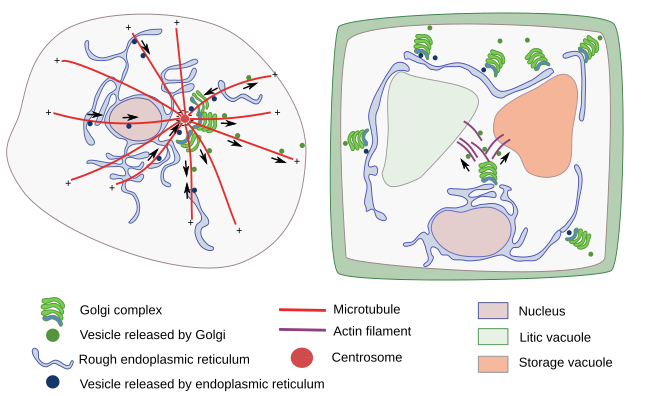
In plant cells, which do not have centrosomes, there are small stacks of cisterns or even individual cisterns scattered throughout the cytoplasm (Figure 3). The Golgi apparatus looks like divided in many units distributed through the cytoplasm. Each of these units work independently. The Golgi apparatus cisterns are smaller than in animal cells, although the total number of cisterns in a plant cell could range from dozens to more than a hundred. In plant cells, the ERGIC (see below) has not been observed, but the trans Golgi network (TGN) is well-developed, so much that some authors endorse it as a different organelle. The TGN is also a target compartment for endocytic vesicles. Individual cisterns or stacks are moved thanks to actin filaments, and are found around the transitional areas of the endoplasmic reticulum, which are those regions where endoplasmic reticulum vesicles are formed. The stack organization does not change during these movements. Vesicles released from the stacks that are targeted to vacuoles are moved by actin filaments too. One more feature of Golgi apparatus organization in plant cells is that cisterns do not disappear during mitosis, as it occurs in animal cells, because Golgi apparatus is needed for synthesizing the new cell wall that separates the two new cells during cytokinesis.
There are distinct features of the Golgi apparatus organization depending on the cell type and species. For instance, the fruit fly cells show a Golgi apparatus organization similar to that of plant cells, although they have centrosome. In yeasts, no piles of cisterns have been observed, and it is thought that cisterns are individual functional units. Furthermore, the lateral connections between adjacent cistern stacks have only been observed in mammalian cells.
2. Organization
The Golgi apparatus is a polarized organelle. Cistern piles are organized in domains: cis, intermediate and trans domains (Figure 4). Intermediate cisterns locates between cis and trans domains. In the cis domain, there is an ongoing addition of new material coming from the endoplasmic reticulum via the ERGIC (endoplasmic reticulum Golgi intermediate compartment). In the trans domain, there is a tubulo-vesicular arrangement of the membranes too referred to as TGN (trans Golgi network), where molecules are distributed into vesicles and tubules in their way toward other cell compartments. Thus, the molecule journey through the Golgi apparatus starts in the cis domain, goes through the intermediate domain, and ends in the trans domain. Concurrently, a recycling flux of molecules enclosed in COP-I coated vesicles is going backward. These vesicles bud off from the lateral part of cisterns and are targeted to previous cisterns in the cis-trans pathway. The Golgi apparatus is in permanent renewing and its organization and size is affected by the trafficking of molecules. It is particularly well-developed in those cells showing an intense secretory activity.

Reflecting the organelle polarization, the resident enzymes in the Golgi apparatus are not distributed randomly, but in a particular distribution in the cis-trans axis. For instance, the cis cisterns contain a higher concentration of N-acetylgalactosamine, the intermediate cisterns more N-acectylglucosamine I transferase, and there is more galactosyltransferase and sialyltransferase in the trans cisterns. Furthermore, the cistern membranes get thicker as they move toward the trans domain. In the cis domain, the cistern membranes are similar to the endoplasmic reticulum membranes, whereas in the trans domain are more similar to the plasma membrane.
The polarity of the Golgi apparatus determines where the vesicles are formed and where they are fetched. In the trans domain, vesicles bud off and are targeted to the plasma membrane (exocytosis) or to late endosomes. It looks like all cisterns can form COP-I coated vesicles that are delivered to other cisterns of the Golgi apparatus in a backward journey, while some of them are targeted to the endoplasmic reticulum. It has been suggested that there are two types of COP-I coated vesicles, those that get fused with cisterns of the Golgi apparatus and those shipped to the endoplasmic reticulum. The cis domain of the Golgi apparatus gets COP-II coated vesicles from the endoplasmic reticulum via the ERGIC, wereas the trans domain of the Golgi apparatus is the target for recycling vesicles coming from the endosomes. The functional difference between the cis and trans domain regarding the vesicle trafficking is a consequence of the differential distribution of membrane proteins involved in forming vesicles or in recognizing and capturing vesicles. The first step in the vesicle recognition is carried out by proteins known as golgins (Figure 5).

In addition to a differential distribution of molecules along the cis-trans axis, it appears to be an uneven molecular distribution in each cistern. It has been suggested that resident enzymes, that is, those that perform their role in the Golgi apparatus, are more concentrated in the center part of the cisterns, while those proteins to be processed and transported to post-Golgi compartments are found more often in the peripheral regions of cisterns.
3. Golgi apparatus models
The mechanism by which molecules travel from the cis to the trans domain is not fully understood yet. For instance, it is unknown if the anterograde transport (toward the trans domain) is an active or a passive process, that is, if these molecules are actively selected, or they are just ignored by COP-I vesicles, which transport cargoes to the cis domain/endoplasmic reticulum. To explain this simultaneous anterograde and retrograde transport of molecules, several models have been proposed. Some models may work concurrently, but some are incompatible between each other. Nonetheless, none can explain all the observations we have nowadays. a) The molecular flux does not disorganize the cis-medial-trans domain, either morphologically, molecular composition, and function. b) Glycosylation of glycoconjugates is an ordered line of steps to build the carbohydrates trees. It means that there are a sorting of enzymes in the cis-trans axis. c) Proteins can move between cisterns, and their asymmetrical distribution is more a consequence of fast movements than confinement. d) In animal cells, there are tubules connecting two cisterns. e) Small molecules may cross the Golgi apparatus very fast, about 2 minutes, while other larger molecules, such as procollagen, may last 15 min.
a) Cisternal maturation model (Figure 6). It poses that ERGICs get fused between each other to form the first cistern at the Golgi cis domain. Then, this cistern moves toward the trans domain, where it is eventually split into vesicles targeted to other cell compartments. The internal material of the cistern is progressively processed during this journey, i.e., there is a maturation of the cistern. Currently, this is the most accepted model because it can explain some observations that do not fit in other models. For instance, procollagen proteins are about 300nm long and do not fit in common vesicles, so they cannot travel from cistern to cistern in vesicles, but they can be moved within cisterns.In this model, there is retrograde transport mediated by COP-I vesicles that return proteins to previous cisterns or to the endoplasmic reticulum. Another way to maintain the molecular asymmetry in the cis-trans axis is mediated by the cistern membrane thickness. As mentioned above, the membranes of the cis cistern are thinner than those of the trans domain. Some proteins may "feel" more comfortable in a membrane as thick as their transmembrane domain length. The cisternal maturation model explains very well the Golgi flux in yeasts.

In animal cells, a Golgi stack may be surrounded by more than a thousand of COP-I vesicles. Two types of COP-I vesicles have been found according to their molecular composition and appearance. One of them may work for retrograde transport between cisterns and the other one for recycling between the Golgi apparatus and the endoplasmic reticulum. The efficiency for fetching molecules by COP-I vesicles may differ for different molecules so that this may be a mechanism for a differential distribution of molecules in the Golgi apparatus domains. An interesting observation is that Golgi apparatus resident proteins have found in COP-I vesicles.
b) Vesicular transport or stationary cistern model. In this model, ERGIC and vesicles coming from the endoplasmic reticulum are fused with the first cis cistern. From this cistern, vesicles bud off and fuse with the next cistern of the Golgi stack, and so on. In this way, the molecules are transported by vesicles from one cistern to the next, while they are specifically processed in each cistern. The support for this model has declined nowadays.
c) Connection by tubules. Tubular connections between adjoining cisterns have been observed at electron microscopy. These connections are like bridges, which appear to be transient and depending on the material to be processed. This proposal is not incompatible with the other models, since tubular connection may happen in both stable cisterns and between cisterns that move through the stack.
The cisternal maturation model does not explain how small molecules may be faster than larger ones. If they are within a cistern, they should travel at the same speed. The tubular connection may solve this question because the tubule allows to only the small molecules to jump from a cistern to the next. However, these tubules have only been observed in mammals, not in plants or yeasts. In addition, these connections may break the Golgi apparatus polarity because, by diffusion through the tubules, molecules could go backward.
4. Functions
Many biochemical processes are done in the Golgi apparatus, such as glycosylation, phosphorylation, sulfaction and proteolytic reactions. Furthermore, it is a sorting compartment to ship molecules for secretion and to other cell compartments, included endosomes and lysosomes, as well as the plasma membrane.
Glycosylation
The Golgi apparatus is a major glycosylation center of the cell. Many carbohydrates that form part of glycoproteins, proteoglycans, glycolipids and other polysaccharides, such as hemicellulose in plants, are synthesized, linked and modified in the Golgi apparatus. It is estimated that about 85 % of the secreted proteins are glycosylated. The synthesis of many of these carbohydrates begins in the endoplasmic reticulum and are modified and enlarged in the Golgi apparatus. Sialic acid is a carbohydrate specifically added in the Golgi apparatus. Polypeptides are linked to the carbohydrate part by two types of bonds. a) N-glycosilation takes place in the endoplasmic reticulum, and the linked carbohydrates are enriched in manose. This chains are modified and lengthened in the Golgi apparatus. b) In the Golgi apparatus, carbohydrates are linked to the peptidic part through a particular type of binding known as O-type glycosylation, which is the attachment of carbohydrates to the hydroxyl groups of amino acids, such as serine, threonine and hydroxylysine. This type of bond is present in proteoglycans. Sulfaction of proteoglycans also happens in the Golgi apparatus, as well as phosphorylation, palmitoylation, methylation, and other chemical modifications. In plants, the glycosylation function of the Golgi apparatus is essential because many glycoconjugates are part of the cell wall. However, cellulose is synthesized at the cell membrane.
Carbohydrates are added by glycosyltransferases and removed by glycosilases. About 200 different types of these enzymes can be found in the Golgi apparatus. Different cisterns have different roles in the carbohydrate processing, which is an ordered process. Evidences suggest that there is a concentration gradient of enzymes, from cis- to trans-domain, so that those enzymes involved in the first steps of glycosylation are concentrated on the cis domain, and those participating in the last steps are mostly found near the trans domain. The synthesis of polysaccharides is quite different from polypeptide or DNA synthesis. Polypetides and DNA needs only a few enzymes and are assembled by using a template. Carbohydrates need a enzyme for each step of synthesis, which works in a particular moment during the complex chain of reactions. For it is a very complex and expensive process, the glucidic component of proteins, lipids and free carbohydrates has to be extremely important for the cell.
The synthesis of biglycan and decorin proteoglycans are examples of asymmetrical distribution of enzymes along the cis-trans axis. Both proteoglycans consists of a polypeptide chain linked to either a chain (decorin) or two chains (biglycan) of glycosaminoglycans, respectively. The glycosaminoglycans are sequentially added to the polypeptide. First, in the cis domain, a xylose tetrasaccharide is the starting point of the carbohydrate chain. In the intermediate and trans domains, more than 100 hundred of disaccharide pairs are polymerized. At the same time, sulfate groups are added to the growing chain.
Synthesis of sphingolipids
Tthe synthesis of sphingomyelins and glycosphingolipids is finished in the Golgi apparatus. Ceramide, which is synthesized in the endoplasmic reticulum, is the molecular base used by enzymes located in the Golgi apparatus for building up these lipid types. Much attention have been paid to sphingolipids because, together with cholesterol, they are thought to be involved in the lateral heterogeneity of the plasma membrane. It means that microdomains with differential functions may exist in the plasma membrane. VLDL (very low density lipoproteins) are apolipoproteins also assembled in the Golgi apparatus.
Sorting comparment
The Golgi apparatus is a center for sorting and shipping molecules, which are coming from the endoplasmic reticulum or synthesized in the Golgi apparatus itself. Once processed, these molecules are sorted into different types of vesicles and targeted to other cell compartments. The TGN is the structure where most of this process takes place (see figure).
a) Some vesicles are targeted to the plasma membrane, a process known as exocytosis. There are two types of exocytosis: constitutive and regulated. Some cells have an apical domain and a baso-lateral domain. They are polarized cells and the plasma membrane needs to get different molecular sets depending on the cell domain. The Golgi apparatus sends specific molecules to each domain, contributing in this way to establish and maintain the cell polarity.
b) Furthermore, there are vesicles leaving the trans domain and targeted to late endosomes/multivesicular bodies/lysosomes, or to vacuoles in plants.
c) From the TGN, recycling vesicles are targeted to previous cisterns of the Golgi stack. The trans domain also gets vesicles coming from endosomes as a recycling pathway. In plants, the Golgi apparatus can fetch vesicles directly coming from the plasma membrane. Thus, this domain of the Golgi apparatus may participate in both, exocytosis and endocytosis.
Cargo selection
A variety of vesicles with different morphologies arises from the tubular expansions of the TGN membrane complex, not just typical round-shape vesicles. Molecules must be selected and included in specific vesicles to be shipped to different targets. Several mechanisms are able to sort molecules depending on their final place in the cell. There are even different ways to select molecules targeted to the same compartment. Those transmembrane proteins targeted to late endosomes (and basolateral membranes in epithelial cells) are selected by a particular amino acid sequence of their cytosolic domain. This sequence is recognized by an adapter proteins, which are concentrated in a restricted area where clathrin coated vesicles are formed. However, acid hydrolases, soluble enzymes targeted to late endosomes/lisosomes, are selected by having a 6-phosphate-manose, which is specifically recognized by a receptor and packaged in vesicles.
Molecules targeted to the plasma membrane (or to the apical membrane of the epithelial cells) are selected differentially depending on the type of exocytosis: constitutive or regulated. Many are selected by selectins, proteins that recognize type O and N glycosyl bonds. Others, such as lipase lipoprotein, are sorted by their affinity with proteoglycans, that in turn have affinity for membrane domains with high density of sphingomyelins. In fact, vesicles enriched in sphingomyelin and lipase lipoproteins have been observed.
The cytoskeleton moves vesicles to their target compartment. For example, in animal cells, microtubules transfer vesicles to the plasma membrane, whereas in plant cells, vesicles targeted to vacuoles are propelled along actin filaments.
Non conventional
The Golgi apparatus performs other non-conventional functions. It works as a calcium storage, as an intracellular signaling platform, contributes to the cellular levels of sterols, starts the cell response to starvation, it is a microtubule nucleator, and it influences the expression of some genes.
-
Bibliography ↷
-
Bibliography
Farquhar, MG, Palade GE. 1981. The Golgi apparatus (Complex) - (1954-1981) - from artifact to center stage. The journal of cell biology. 91: 77s-173s.
Glick, BS, Luni A. 2011. Models for Golgi traffick: a critical assessment. Cold Spring Harbor perspectives in biology. 3: a005215.
Hellicar J, Stevenson NL, Stephens DJ, Lowe M. 2022, Supply chain logistics – the role of the Golgi complex in extracellular matrix production and maintenance. Journal of cell sciences. 135: jcs258879. doi:10.1242/jcs.258879.
Vildanova MS, Wang W, Smirnova EA. 2015. Specific organization of Golgi apparatus in plant cells. Biochemistry (Moscow). 79: 894-906.
Witkos TM, Lowe M. 2017. Recognition and tethering of transport vesicles at the Golgi apparatus. Current opinion in cell biology. 47:16–23.
-